Creation of elements
How elements are created? The answer is in the starts. Let’s have a look to the creation of what we are made of.
Elements are formed through a process called nuclear fusion. Nuclear fusion is the process by which atomic nuclei come together to form a heavier nucleus. Remember that we are talking about nuclear fusion, not nuclear fission. Nuclear fission is the process by which an unstable heavy nucleus breaks down into a smaller nucleus, releasing energy, but we are talking about nuclear fission here. For example, in nuclear fusion, 2 atoms of hydrogen fuse together to form an atom of helium and release a considerable amount of energy. But there is an inconvenience: the nuclei of hydrogen (or any other elements) are positively charged and need to be forced to join. It is not an easy task that requires a considerable amount of energy and pressure. However, once the nuclear fusion starts, releases more energy than was required to initiate it (at least for elements lighter than iron). Humans, for the moment, cannot create nuclear fusion as a source of energy (but scientists are working on it). Nonetheless, the pressure and the temperature reached inside stars give the conditions for nuclear fusion to happen, in fact, the light and heat that the stars produce are consequences of nuclear fusion, and if it was not for nuclear fusion, we will not have stars as we know them. The great majority of elements have been (and are being made now) in the stars via nuclear fusion. It will help us look at the stars’ life cycle before we see the creation of elements.
Stars
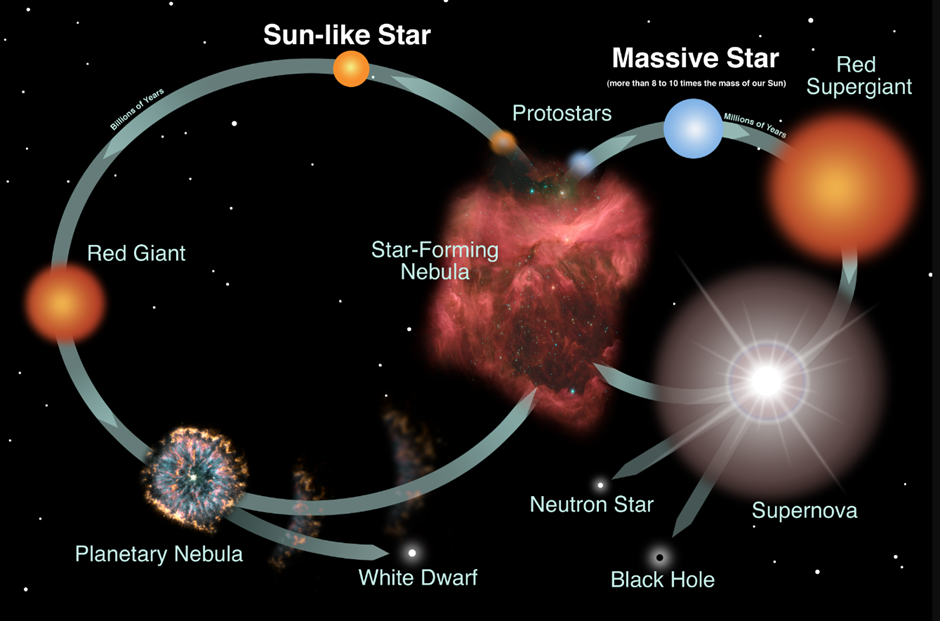
Stars are made of ionized gas called plasma. Stars are born due to clouds of dust that coalesce due to gravitational forces and are called nebulae, an example is the Orion nebula. Over time, the cloud collapses under its gravitational forces and the centre starts to heat up. When the centre of the nebula reached a critical mass, the heat and pressure generated in the centre are high enough to initiate nuclear fusion, and the star is born.
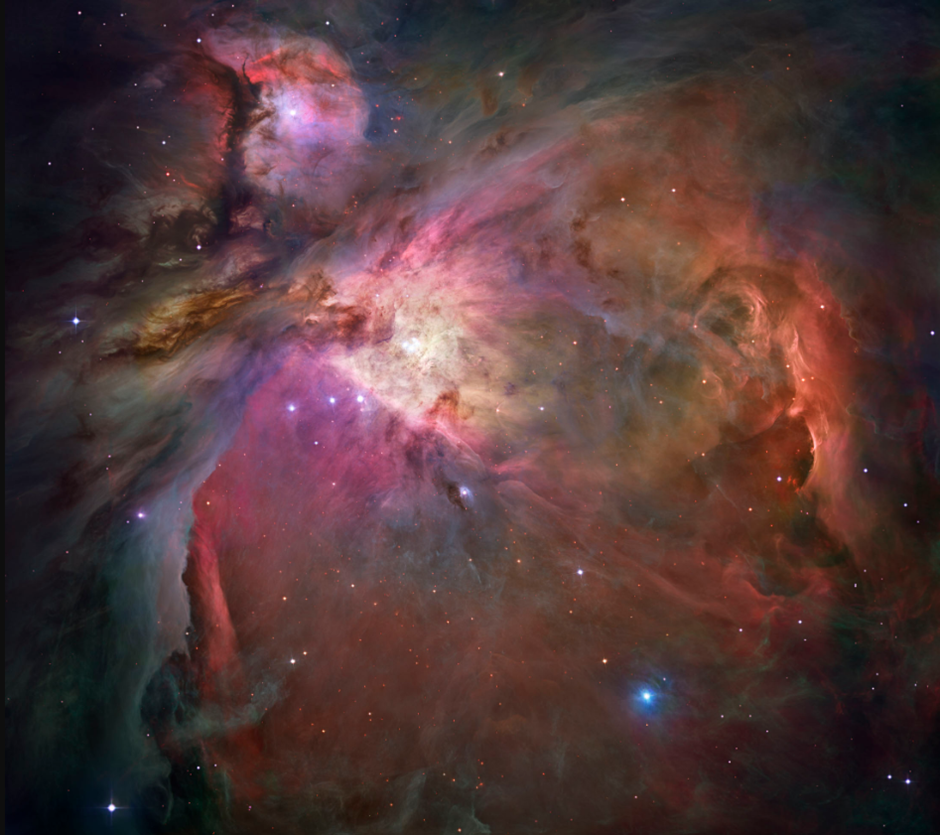
In the stars, we have 2 competing forces acting:
- the gravity force due to its mass. This force pulls the start inwards (or in other words, tries to collapse the start within itself) and
- pressure due to the energy of the core generated by nuclear fusion. This force pushes the star outwards (this force tends to expand the star).
The equilibrium between these 2 forces is called hydrostatic pressure and, as long as they ear equal, we can consider that the star is stable.
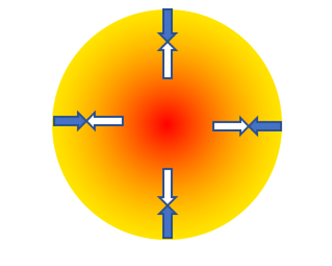
At some point, the star will run out of hydrogen to maintain the nuclear reactions, a star like the sun, will require around 50 billion years to reach this point. When the hydrogen is consumed, the gravity force will shrink the core of the star, increasing the pressure and temperature in its core, and the helium will now be used to produce carbon via the triple-alpha process. While the core collapses, the outer layers of material in the star expand and form what is called a red giant (or supergiant). However, depending on the mass of the star, the evolution and longevity of the star will vary.
- When a medium-sized star (up to about 7 times the mass of the Sun) reaches the red giant phase of its life, the core will continue using helium to form carbon and keep the star alive for some time. Once the helium in the core is gone, the star will shed most of its mass, forming a cloud of material called a planetary nebula. The core of the star will cool and shrink, leaving behind a small, hot ball called a white dwarf. A white dwarf doesn’t collapse against gravity because of the pressure of electrons repelling each other in its core. The white dwarf radiates its remaining heat into space for many billions of years. (Note: here I use the English definition of billion, it is equivalent to the European 1,000 million). In the end, it will just sit in space as a cold dark mass sometimes referred to as a black dwarf.
- Stars with more than 7 times the mass of the Sun follow similar steps to the medium-mass stars but form what are called red supergiants. When the supply of helium runs out, the core will contract again, but since the core has more mass, it will become hot and dense enough to produce heavier elements, up to iron. Up to this point, the energy from the fusion reactions allows the start to fight gravity. However, fusing iron requires an input of energy, rather than producing excess energy. The core temperature rises to over 100 billion degrees as the iron atoms are crushed together. The repulsive force between the positively-charged nuclei overcomes the force of gravity, and the core recoils out from the heart of the star in an explosive shock wave producing a tremendous explosion called a supernova. About 75% of the mass of the star is expelled into interstellar space. What happens to the core? It depends on the mass of the star:
- If the left core is about 1.4 to 5 times the mass of the sun, it will collapse into a neutron star. Neutron stars also have powerful magnetic fields which can accelerate atomic particles around their magnetic poles producing powerful beams of radiation. Those beams sweep around like massive searchlight beams as the star rotates. If such a beam is oriented so that it periodically points toward the Earth, we observe it as regular pulses of radiation that occur whenever the magnetic pole sweeps past the line of sight. In this case, the neutron star is known as a pulsar.
- If the core is larger than 5 times the mass of the star, it will collapse into a black hole.
Elements
We have seen a general evolution of the stars and how some elements are formed. We are going to go into a bit more detail about how the elements are formed in space. It will be useful to have a periodic table with the origin of the elements, such as:
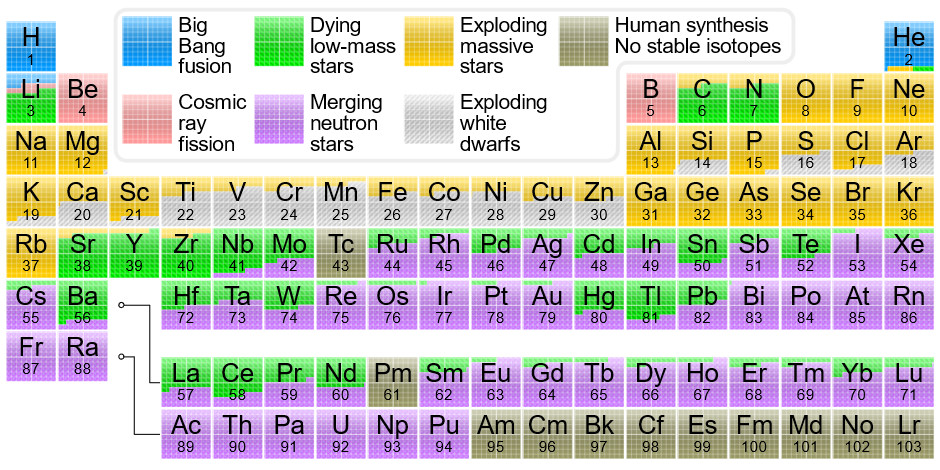
The first elements (hydrogen, helium and a small portion of lithium) were formed as a consequence of cooling from the big bang. It is estimated that the big bang occurred around 13.8 billion years ago (or 13,800 million years if we use the European format). The hydrogen and helium nuclei formed between 3 and 20 minutes of the big bang. However, radiation and heat were intense at those earlier stages and the nuclei were unable to retain electrons and form neutral atoms. It took around 380,000 years for the universe to cool down enough to allow the electrons to stay in orbit around atomic nuclei.
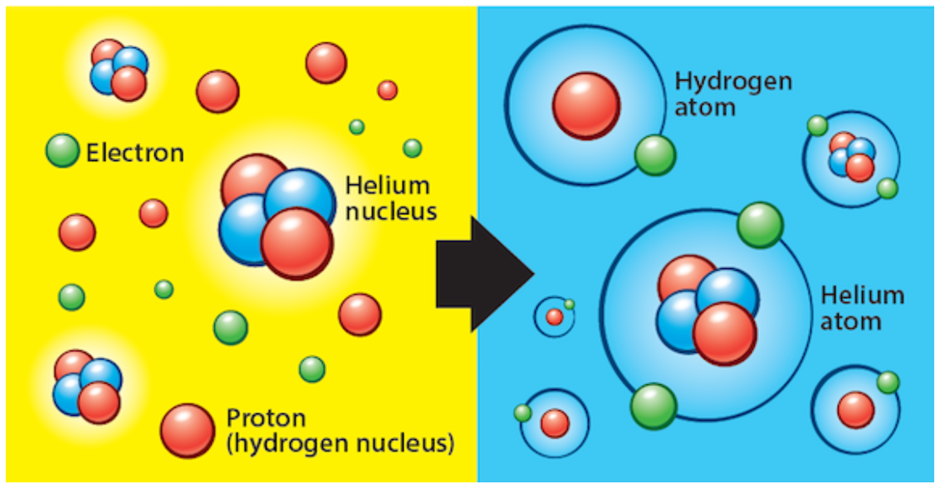
When the 1st stars formed, the fusion of hydrogen to form helium started:
4 (1H) => 4He + 2 e+ + 2 neutrinos + energy
Or in more detail:
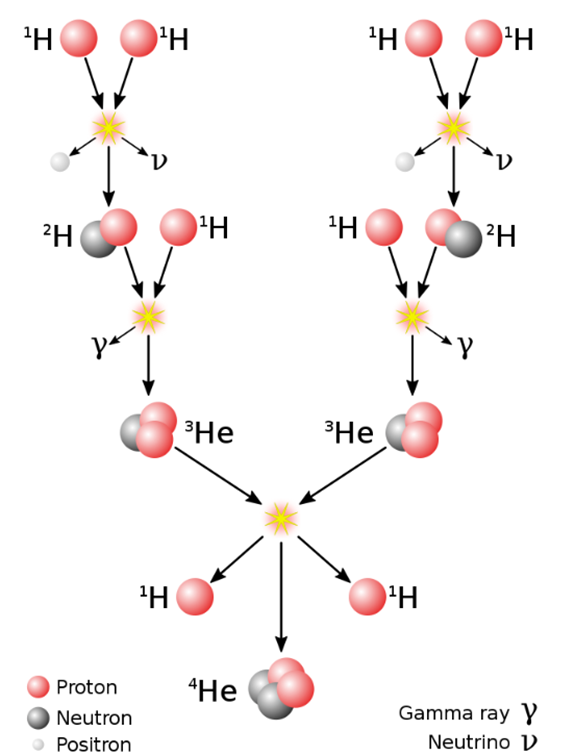
Once the hydrogen is consumed, the nuclear fusion stops, the core of the star collapses under the gravity force, increasing its pressure and temperature and the next stage of the star commences (red giant or red supergiant) with the helium starting to fusion to form carbon via the triple-alpha process:
3 (4He) → 12C + energy
Or in more detail:
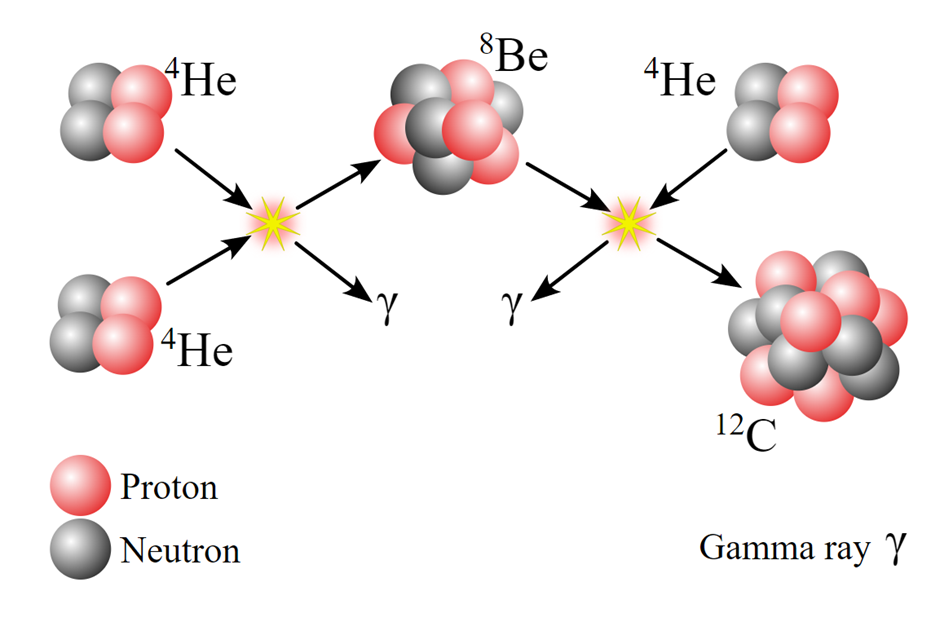
In the red supergiants, other reactions occur, giving birth to heavier elements:
12C + 12C → 24Mg + energy
4He + 12C → 16O + energy
4He + 16O → 20Ne + energy
16O + 16O → 32S + energy
12C + 12C → 24Mg + energy
16O + 16O → 28Si + 4He
28Si + 7(4He) → 56Ni + energy → 56Fe
To form heavier elements than iron is via neutron capture: in this process, neutrons are absorbed by the atomic nuclei of lighter elements, increasing their atomic number and transforming them into heavier elements. This process can occur in two ways: slow neutron capture (also known as s-process) and rapid neutron capture (also known as r-process).
- Slow neutron capture: In the slow neutron capture process, the neutron is absorbed slowly over time, allowing the nucleus to undergo beta decay and stabilize before another neutron is absorbed. This process is responsible for the formation of about half of all the elements heavier than iron in the universe, including uranium.
- Rapid neutron capture: In the rapid neutron capture process, the neutron is absorbed quickly, resulting in a highly unstable nucleus. This instability can lead to further neutron capture or beta decay, producing even heavier elements. The r-process is responsible for the formation of elements heavier than iron, including elements such as gold, platinum, and uranium.
It is believed that the slow neutron capture process occurs in the cores of low- to intermediate-mass stars, while the rapid neutron capture process occurs in the aftermath of supernova explosions, where the intense heat and pressure of the explosion can provide the conditions necessary for rapid neutron capture to occur.
There are 3 light elements that when formed in the stars, break down to helium, as they are unstable at high temperatures. These elements are lithium, beryllium and boron. How are they formed? It is ‘generally’ accepted that they are formed by the collision of cosmic rays with hydrogen and helium in the interstellar space (in the next paragraph why I say ‘generally’). Cosmic rays are atoms and subatomic particles that are accelerated to near the speed of light. When cosmic rays hit atoms, they produce new elements. During its journey across the galaxy, a cosmic ray may hit an atom of hydrogen or helium in interstellar space. Since the cosmic ray is travelling so fast, it will hit with great force, and part of its nucleus can be “chipped off.” For example, the nucleus of a carbon atom in the outer layers of a large star may be accelerated to near light speed when the star explodes as a supernova. The carbon nucleus (which we now call a cosmic ray) flies through space at a high speed. Eventually, it collides with a hydrogen atom in an open space. The collision fragments the carbon nucleus, which creates two new particles: helium and lithium.
Why did I write ‘generally’ above? Well, scientists are constantly trying to explain the universe as we know it and they are constantly reviewing what we know. For example, they have realised that the amount of lithium in the universe explained by the collision of cosmic rays could not account for the lithium observed. In May of 2020, a team led by Sumner Starrfield of Arizona State University published an article in The Astrophysical Journal explaining that the formation of lithium was produced in what is called a ‘classical nova’ A classical nova happens when a white is orbited by a larger star. Gas falls from the larger star onto the white dwarf and when enough gas has accumulated on the white dwarf, an explosion, or nova, occurs.
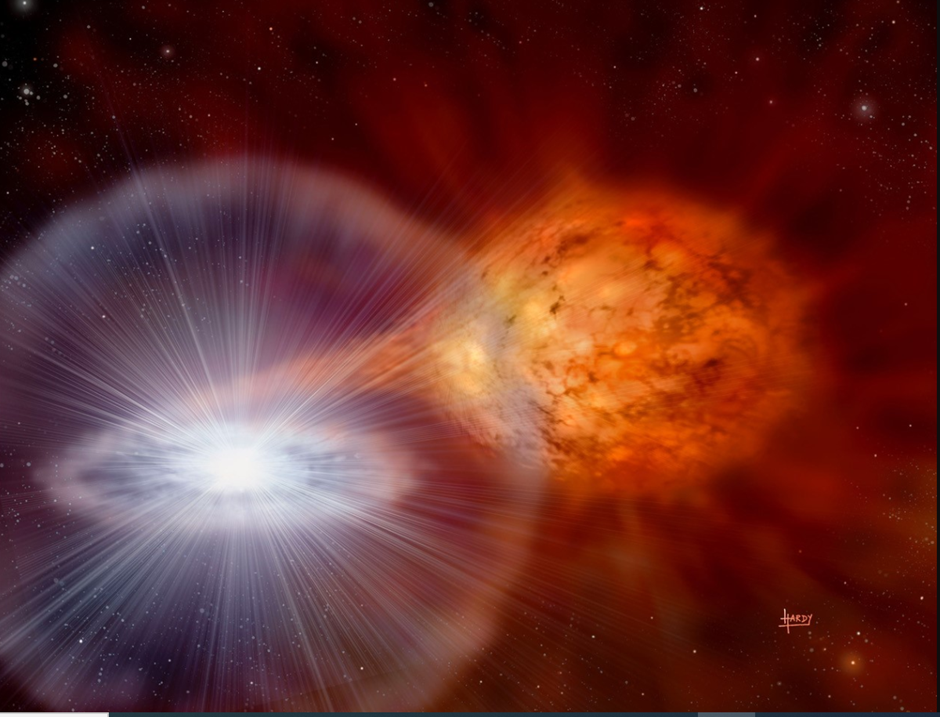
Credits: Illustration by David A. Hardy. Source: https://www.nasa.gov/feature/lithium-comes-from-exploding-stars
Final remarks
I have tried to give a quick overview of the formation of the stars. It is a very fascinating topic and scientists are constantly reviewing and modifying theories as we have more information about the world around us and the universe.
I hope that you have liked this post. If you have any comments or questions, please, add them below. It will help me with future posts and other people who read them.
Sources
http://imagine.gsfc.nasa.gov/docs/teachers/elements
https://en.wikipedia.org/wiki/Nuclear_fusion
https://en.wikipedia.org/wiki/Stellar_nucleosynthesis
https://imagine.gsfc.nasa.gov/science/objects/stars1.html
https://science.nasa.gov/astrophysics/focus-areas/how-do-stars-form-and-evolve
Nuclear Reactions In Stars (secretsofuniverse.in)
https://en.wikipedia.org/wiki/Triple-alpha_process
https://imagine.gsfc.nasa.gov/educators/elements/imagine/07.html
https://astronomy.com/magazine/ask-astro/2018/12/the-first-element
https://kids.frontiersin.org/articles/10.3389/frym.2019.00092
https://www.nasa.gov/feature/lithium-comes-from-exploding-stars