Questions 1 and 2 are in OCR, AS Level Chemistry, 2023, H032/02 Depth in chemistry. Questions 1 and 2 –
If you find this useful, please leave a comment at the end of the page.
A link to a video of this paper can be found in https://youtu.be/tLww3fSvGH8
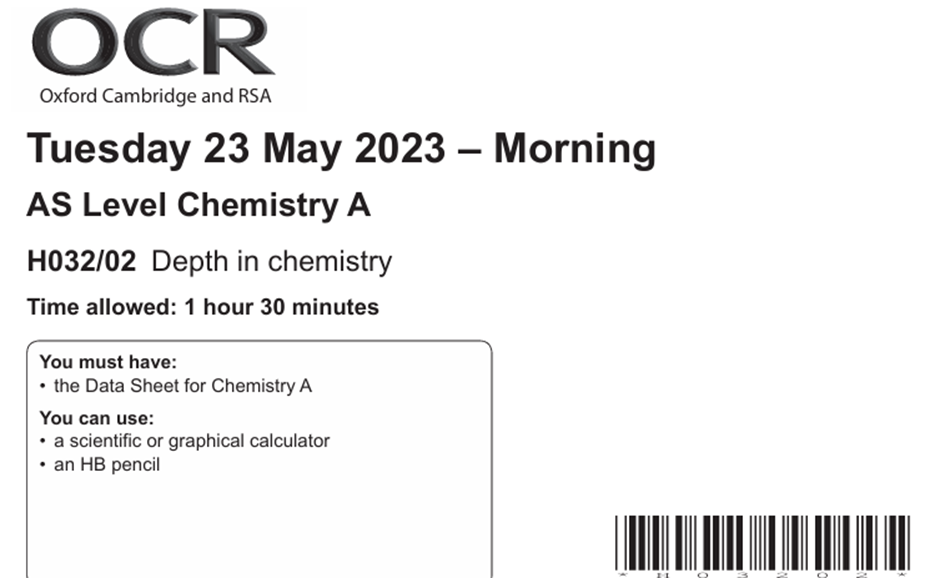
3
This question is about halogens and halogen compounds.
(a)*
Seawater contains very small quantities of dissolved iodide ions.
The concentration of potassium iodide, KI, in seawater is 0.150gdm–3.
Iodine can be extracted by bubbling chlorine gas through seawater.
Explain why chlorine is more reactive than iodine and determine the volume, in dm3, of seawater that is needed to manufacture 1.00 tonne of iodine, I2.
[6]
When chlorine gas (Cl2) is bubbled through seawater, it displaces iodine from iodide ions (I−) due to chlorine’s higher reactivity. The reaction is as follows:
2I− + Cl2 → 2Cl− + I2
(Observation: The solution may turn brown due to the formation of iodine.)
Chlorine is more reactive than iodine due to several factors:
- Effective Nuclear Charge: Chlorine has smaller size and higher effective nuclear charge meaning that it can gain electrons more easily.
- Electronegativity: chlorine has a higher electronegativity compared to iodine, meaning chlorine has a greater tendency to attract electrons towards itself.
- Atomic Size: chlorine has a smaller atomic radius, resulting in a stronger attraction between the nucleus and the outermost electrons.
In summary, chlorine’s higher electronegativity, smaller atomic size, and stronger effective nuclear charge make it more reactive than iodine, enabling it to attract and gain electrons more readily.
The calculation of the volume, in dm3, of KI needed to produce 1.00 tons of I2 involves a chain of units conversions and moles proportions. There are different ways to solve this problem. In my calculations I use the Factor label method
First, I calculate the molar masses of I2 and KI
- M(I2) = 126.9 × 2 = 253.8 g/mol
- M(KI) = 29.1 + 126.9 = 166 g/mol
From the stoichiometry of the reaction, we have that for each mol of I2, we need 2 moles of KI
2I− + Cl2 → 2Cl− + I2
Therefore, for 253.8 g of I2, we are going to need 166 × 2 = 332 g of KI.
As 1.00 tone of I2 is 1,000,000 g of I2, this is equivalent to:
$$ \small 1,000,000 \, \text{g I}_2 \times \left( \frac{332 \, \text{g KI}}{253.8 \, \text{g I}_2} \right) = 1.308 \times 10^6 \, \text{g KI} $$
And with a concentration of 0.150 g/dm3, we need:
$$ \small 1.308 \times 10^6 \, \text{g KI} \times \left( \frac{1 \, \text{dm}^3}{0.150 \, \text{g}} \right) = 8.721 \times 10^6 \, \text{dm}^3 $$
Using 3 significant figures: 8.72 × 106 dm3 of sewater.
(b)
Chlorine reacts with calcium hydroxide to form Ca(OCl)2, which is the active ingredient in bleaching powder.
2Cl2 + 2Ca(OH)2 → CaCl2 + Ca(OCl)2 + 2H2O
This is a disproportionation reaction.
State what is meant by disproportionation and use oxidation numbers to show that disproportionation has taken place.
Disproportionation (or dismutation) is a redox reaction where one compound is oxidised and reduced in the same reaction.
To explain the disproportionation we need to refer to the oxidation numbers. Let’s find these numbers:
- Cl in Cl2 has an oxidation number 0 as it is in its natural form.
- Cl in CaCl2. The Ca is in oxidation state +2, as the CaCl2 is neutral, both atoms of Cl compensate the +2 of the Ca, making the oxidation number of the Cl -1.
- Cl in Ca(OCl)2. The Cl is in the form of ClO–. The O is in oxidation state -2, as the ion ClO– has a charge -1, the Cl only compensate 1 of the negative charges of the O, making the oxidation number of the Cl +1.
This is a disproportionation reaction because the Cl with oxidation number 0 in the in Cl2 has been reduced to oxidation number -1 in the CaCl2 and oxidised to oxidation number +1 in the Ca(OCl)2.
[3]
(c)
A student is studying the hydrolysis of haloalkanes.
The equation for the alkaline hydrolysis of 2-bromopropane, CH3CHBrCH3, is shown below.
CH3CHBrCH3 + OH– → CH3CHOHCH3 + Br –
Use the curly arrow model to outline the mechanism for the alkaline hydrolysis of 2-bromopropane.
Show relevant dipoles and lone pairs, and name the mechanism.
Many of the reactions of halogenoalkanes are nucleophilic substitutions (SN). Depending on the structure, the mechanism can be SN1 (tertiary halogenoalkanes) or SN2 (primary halogenoalkanes). Secondary halogenoalkanes can proceed through a combination of both SN1 and SN2 reactions depending on their specific structure. The marking scheme allows SN1 and SN2 mechanism in this exercise.
For SN2:
- Nucleophilic substitution reactions rely on the polarization of the carbon-halogen bond. This occurs because the halogen is more electronegative than the carbon atom, resulting in the carbon atom carrying a partial positive charge. Consequently, the electron pair in the carbon-halogen bond is pulled closer to the halogen atom.
- The hydroxide ion (OH−) acts as a nucleophile and attacks the carbon atom bonded to the bromine. A curly arrow indicates the movement of the lone pair of electrons from the hydroxide ion to the carbon atom, forming a new bond.
- The bond between carbon and bromine is breaking while the bond between carbon and oxygen is forming.
- The bromide ion (Br−) leaves, taking its bonding electrons with it. A curly arrow shows the movement of the bonding electrons from the carbon-bromine bond to the bromine atom.
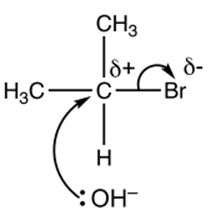
For SN1:
- The first step is the dissociation of the carbon-bromine bond in 2-bromopropane. The bromine atom leaves, taking the bonding electrons with it, and forms a bromide ion (Br−). This step is slow and results in the formation of a carbocation (an intermediate with a positive charge) at the carbon previously bonded to bromine.
- The hydroxide ion (OH–) then attacks the carbocation. The lone pair of electrons on the hydroxide ion is donated to the positively charged carbon, forming a new bond.
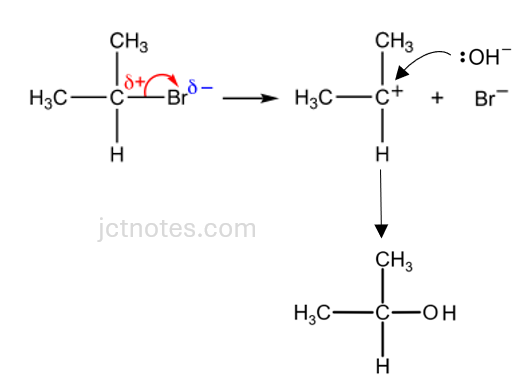
name of mechanism …………nucleophilic substitution……………………………………….
[3]
(d)
The student sets up an experiment to compare the rates of hydrolysis of 2-bromopropane and 2-iodopropane.
The student uses the method below.
Step 1
Place two test tubes, both containing aqueous silver nitrate and ethanol, in a water bath at 60°C.
Step 2
Add five drops of 2-bromopropane to one test tube and five drops of 2-iodopropane to the other test tube.
Step 3
Record the time taken for a precipitate to appear in each test tube.
(i)
Complete the table below to show the formula and colour of each precipitate formed.
Haloalkane | Formula of precipitate | Colour of precipitate |
---|---|---|
2-bromopropane | ||
2-iodopropane |
[2]
As seen in the previous question, in the hydrolysis, the student is replacing the halogen by a hydroxyl group via nucleophilic substitution:
CH3-CHX-CH3 + OH– → CH3-CHOH-CH3 + X–
The addition of aqueous silver nitrate precipitates the relevant silver halide:
X– + Ag+ → AgX↓
This is a typical identification reaction of halogens. The colour of the precipitate and its solubility in ammonia allows to differentiate between them:
Silver halide | Colour | Solubility in dilute ammonia | Solubility in concentrated ammonia |
---|---|---|---|
AgCl | White | Soluble | Soluble |
AgBr | Cream | Insoluble | Soluble |
AgI | Yellow | Insoluble | Insoluble |
Therefore, the table can be completed as:
Haloalkane | Formula of precipitate | Colour of precipitate |
---|---|---|
2-bromopropane | AgBr | Cream |
2-iodopropane | AgI | Yellow |
(ii)
Predict which precipitate would form first and explain the difference in the rates of hydrolysis of 2-bromopropane and 2-iodopropane.
The experiment looks at how easily the bonds between carbon (C) and the halogen (X) in 2-bromopropane and 2-iodopropane break. The bond strength depends on the size of the halogen atom: larger atoms make weaker bonds. Iodine is larger than bromine, so the carbon-iodine (C-I) bond is weaker than the carbon-bromine (C-Br) bond. Because of this, the C-I bond breaks more easily, meaning that 2-iodopropane undergoes hydrolysis faster than 2-bromopropane. As a result, the yellow precipitate of silver iodide (AgI) will form first.
[1]
4
This question is about the enthalpy change of combustion of alcohols.
(a)
Explain the term enthalpy change of combustion.
The standard enthalpy change of combustion (ΔcHO) is the heat energy released when one mole of a substance undergoes complete combustion in excess of oxygen under standard conditions (25 °C, 100 kPa pressure), with all reactants and products in their standard states.
(b)
A student carries out an experiment to determine the enthalpy change of combustion, ΔcH, of butan-1-ol, CH3CH2CH2CH2OH.
The student sets up the apparatus as shown below.
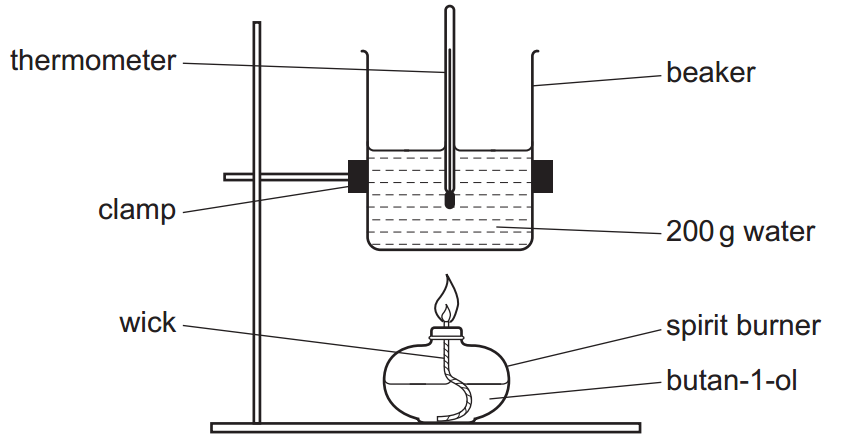
The student’s results are shown in the table below.
Initial temperature of water/°C | 18.5 |
Final temperature of water/°C | 49.5 |
Mass of burner before heating/g | 212.38 |
Mass of burner after heating/g | 211.07 |
(i)
The thermometer had an uncertainty of ± 0.25°C in each temperature reading.
Calculate the percentage uncertainty in the temperature change.
Each temperature reading has an uncertainty of ± 0.25 °C. Since there are two readings (initial and final), the total absolute uncertainty is:
Total absolute uncertainty = 0.25 + 0.25 = 0.50 °C
The students measured the temperature change as follows:
Temperature change = Final temperature − Initial temperature = 49.5 − 18.5 = 31.0 °C
Thus, the percentage uncertainty is calculated as:
$$ \small \text{Percentage Uncertainty} = \frac{\text{Absolute Uncertainty}}{\text{Measured value}} \times 100 = \frac{0.50}{31.0} \times 100 = 1.61\% $$
percentage uncertainty = …………………1.61…………………………… %
[1]
(ii)*
Use the student’s results to determine ∆cH of butan-1-ol in kJmol–1.
Explain why this value of ΔcH is different from the data book value and suggest how the experimental design could be modified to improve the accuracy of the ΔcH value obtained.
[6]
To determine the standard enthalpy change of combustion, we need to calculate the energy released during combustion and apply it to one mole of butan-1-ol. We assume all the energy released in the combustion has been used to heat the water (we will address this assumption when comparing our results to the data book values).
The heat used to heat the water can be calculated using the formula:
q = mcΔT
Where:
– m is the mass of water (200 g as per the drawing),
– c is the specific heat capacity of water (4.18 Jg−1K−1, from the data sheet),
– ΔT is the increase in temperature (31.0 K from the previous question; since we are dealing with temperature increases, the values in °C and K are numerically the same).
Replacing these values, we get:
q = 200 g × 4.18 Jg-1K-1× 31.0 K = 25,916 J = 25.916 kJ
The mass of butan-1-ol consumed is:
212.38 g−211.07 g=1.31 g
The molar mass of butan-1-ol is calculated as follows:
12.0×4+1.0×10+16.0×1=74.0 g/mol
Therefore, 1.31 grams of butan-1-ol is:
$$ \small 1.31 \, \text{g} \times \frac{1 \, \text{mol}}{74.0 \, \text{g}} = 0.0177 \, \text{mol} $$
$$ \small \frac{25.916 \, \text{kJ}}{0.0177 \, \text{mol}} = 1,464 \, \text{kJ/mol} $$
Given the heat released is 25.916 kJ, we can calculate that the standard enthalpy change of combustion of butan-1-ol is:
The difference from the book value can be attributed to several factors:
- Heat Loss: Heat escapes to the surroundings (air, burner, calorimeter) instead of being fully transferred to the water.
- Incomplete Combustion: Butan-1-ol may burn partially, producing CO instead of CO₂, releasing less energy.
- Evaporation of Fuel: Some fuel evaporates before combustion, reducing the actual mass burned.
- Evaporation of Water: Some water evaporates, resulting in less than 200 g.
- Standard Conditions: Data book uses standard conditions (298 K and 100 kPa); the conditions during the experiment may differ.
To improve the experimental values, consider the following modifications:
- Better isolation of the system: Shield to protect from drafts or use a bomb calorimeter.
- Use a lid to prevent water evaporation.
- Use a metal can instead of a glass beaker to allow better heat transmission.
- Use an excess of oxygen.
- Reduce the gap between the burner and the beaker.
- Conduct the experiment under standard conditions (298 K and 100 kPa).
- Increase the precision by using a balance with three decimal places or a digital thermometer.
- Heat for a longer duration to reduce percentage uncertainty in mass and temperature measurements.
(iii)
Another student carries out the experiment in 4(b) using 150g of water in the beaker instead of 200g.
Calculate the mass of butan-1-ol that would produce the same temperature rise as in the experiment in 4(b).
Assume the same heat losses.
As the amount of water has been reduced by 150/200 × 100 = 75%, the amount of butan-1-ol should be 75% of the initial quantity, that is: 1.31×75/100 = 0.9825 gram. Rounded to 2 DP: 0.98 g.
mass of butan-1-ol = ………………………0.98………………………. g
[1]
(c)
The enthalpy change of combustion of ethanol, ΔcH, in the gaseous state can be calculated using average bond enthalpies.

(i)
Use this value of ΔcH and the average bond enthalpies below to calculate the average bond enthalpy of C=O.
Bond | Average bond enthalpy/ kJmol–1 |
---|---|
C–H | +413 |
C–C | +347 |
C–O | +358 |
O–H | +464 |
O=O | +498 |
The average bond enthalpy, DX-Y, is the enthalpy required to break one mole of X−Y bonds in a gaseous molecule under standard conditions. Since breaking bonds requires energy, these values are always positive.
To calculate the enthalpy change of combustion (ΔCH), we use the formula:
$$ \small \Delta_{\text{C}} H = \sum_{\text{Broken}} D_{X-Y} – \sum_{\text{Formed}} D_{X-Y} $$
In this case, we have the following broken bonds:
- 5 C-H bonds in the C2H6O, 5×413 = 2,065
- 1 C-C bond in the C2H6O. 1×347 = 347
- 1 C-O bond in the C2H6O, 1×358 = 358
- 1 O-H bond in the C2H6O. 1×464 = 464
- 1 O=O bond in each O2. As we have 3 oxygens, the contribution is 3×498 = 1,494
- Total due to broken bonds: 2,065 + 347 + 358 + 464 + 1,494 = 4,728 kJ
And bonds formed:
- 2 C=O bonds in each CO2, as we have 2 CO2, the contribution is 2×2×DC=O = 4DC=O
- 2 H-O bonds in each H2O. as we have 3H2O, the contribution is 2×3×464 = 2,784 kJ
- Total due to bonds formed: 4DC=O + 2,784 kJ.
C=O bond enthalpy = ………………+805………………………kJmol–1
[4]
(ii)
Methoxymethane, CH3OCH3, is an isomer of ethanol.
On combustion, methoxymethane, in the gaseous state, produces carbon dioxide an steam.

ΔcH for methoxymethane is more negative than ΔcH for ethanol.
Explain why the ΔcH values are different, in terms of the bonds broken and the bonds formed.
When comparing the two reactions, the only difference is the bonds broken in methoxymethane and ethanol, while the other components (and therefore bonds) are the same. Breaking bonds requires energy (positive value) and forming bonds releases energy (negative value). Therefore, we can conclude that methoxymethane needs less energy to break down than ethanol.
The exam asks for an explanation and not for calculations, therefore, calculations are ignored for marking. For completeness, I have calculated the average energy to break down the methanol and the methoxymethane:
- For methanol:
- 5 C-H bonds: 5×413 = 2,065 kJ
- 1 C-C bond: 1×347 = 347 kJ
- 1 C-O bond: 1×358 = 358 kJ
- 1 O-H bond: 1×464 = 464 kJ
- Total: 3,234 kJ
- methoxymethane:
- 6 C-H bonds: 6×413 = 2,478 kJ
- 2 C-O bond: 2×358 = 716 kJ
- Total: 3,194 kJ
[2]
5
This question is about alkenes.
(a)
A mixture of alkenes is produced when water is eliminated from alcohol A.
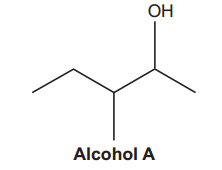
(i)
What is the systematic name of alcohol A?
The longest chain consists of 5 carbon atoms, making it the main chain and naming it as pentane.
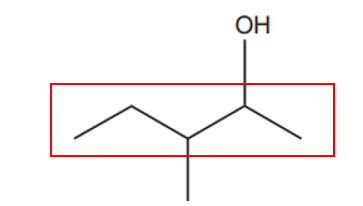
There are two substituents: an alcohol (-OH) and a methyl group (-CH₃). The alcohol group takes precedence (for more details on group priorities, refer to IUPAC nomenclature of organic chemistry – Wikipedia). We number the chain starting from the end closest to the alcohol group, giving the OH group the lowest number, which is 2. This results in the name pentan-2-ol.
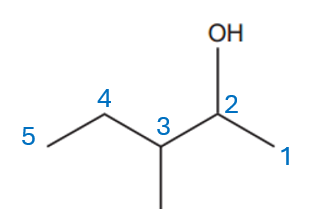
Finally, we add the methyl group to the third carbon, resulting in the name 3-methylpentan-2-ol.
………………………………………………………………………………3-methylpentan-2-ol……………………………………..
[1]
(ii)
Alcohol A is refluxed with an acid catalyst.
- A mixture of alkene isomers B, C and D is formed.
- Alkenes B and C show E/Z isomerism but alkene D does not.
Construct the equation for the formation of alkene D from alcohol A.
Show the structure of the organic product.
The reflux of an alcohol with an acid catalyst produces and alkene, besides, the question mention that they are alkenes. In this reaction, we have that the OH produces a carbon-carbon double bond and releases water. The double bond is going to be formed between the carbon 1 and carbon 2:
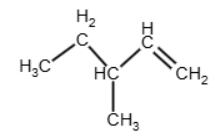
or between the carbon 2 and the carbon 3:
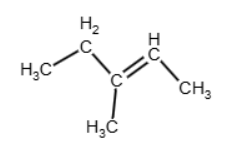
In the case of carbon 2 – carbon 3, we have that each carbon in the double bond has two different groups attached, and it is going to form the E/Z isomers B and C (as it is shown in the table of the next question). Therefore, alkene D is the one with the double bond between carbon 1 and carbon 2, and the reaction is (don’t forget the H2O):
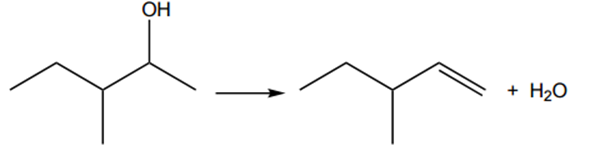
[2]
(iii)
The skeletal formulae of alkenes B and C are shown below.
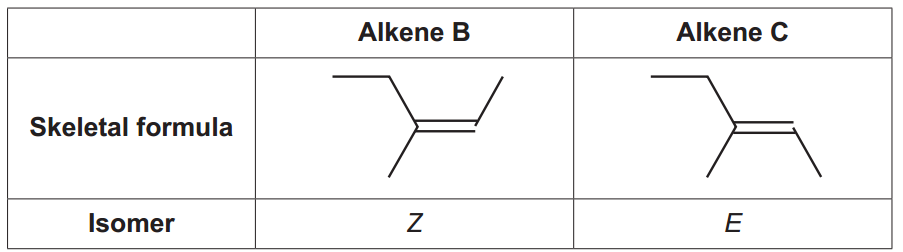
Use the Cahn-Ingold-Prelog priority rules to explain why alkene B is the Z isomer.
The Cahn-Ingold-Prelog priority rules are:
- We compare the atoms directly attached to the double-bonded carbons. The priority is given as follows:
- The higher the atomic number, the higher the priority. For example, C has priority over H.
- If isotopes are attached the isotope with the higher atomic mass takes priority. Say we 12C and 14C, then 14C takes priority.
- If the atoms are the same, we move to the next set of atoms along the chain until a difference is found.
- Multiple bonds are treated as if the bonded atoms are duplicated or triplicated. For example, a carbon-carbon double bond (C=C) is considered as if there are two single bonds to the same carbon, and a carbon-carbon triple bond (C≡C) is treated as if there are three single bonds.
The Z or E designation is given as follows:
- Z (from the German word “zusammen,” meaning “together”) if the higher priority groups are on the same side of the double bond.
- E (from the German word “entgegen,” meaning “opposite”) if the higher priority groups are on opposite sides of the double bond.
To see the priorities, I expand the bonds in alkene B and number the carbon atoms (I label one of the carbons as 3’ to refer to it in the explanation below):
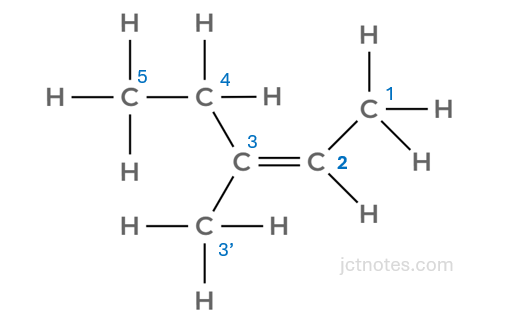
Starting with carbon 2, we have that it is attached to the carbon 1 and to a hydrogen. As the carbon has higher atomic number, it takes priority.
We continue with carbon 3. It is attached to 2 carbons, both have the same atomic number, we move to the next carbon on each side: carbon 4 and carbon 3’:
- Carbon 4 is attached to a carbon (carbon 5) and to a hydrogen.
- Carbon 3’ is attached to 3 hydrogens
At this point, we have that the carbon number 5 has priority over the hydrogens (as it has higher atomic number), then the priority is in the branch of the carbon 5.
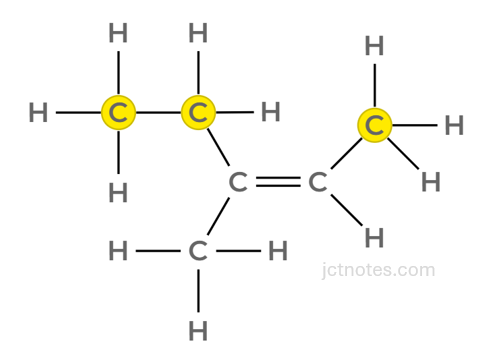
In a nutshell: the priorities are given by the higher atomic numbers of the atoms attached to the carbons of the double bond. In the case of alkene B, the highest priority groups (CH3– and CH3-CH2-) are in the same sideCH3– and CH3-CH2-) are in the same side, it is an Z isomer.
[2]
(b)
A chemistry company is developing water-soluble polymers.
The chemists decide to use compound E, shown below, as the monomer.
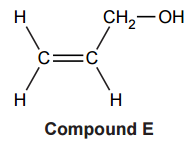
(i)
Draw a section of the polymer formed, showing two repeat units, and suggest why this polymer is likely to be soluble in water.
Section of polymer (two repeat units): This is an addition polymerisation, where the double bond in the monomer breaks and forms new bonds with adjacent monomers, creating a polymer chain:
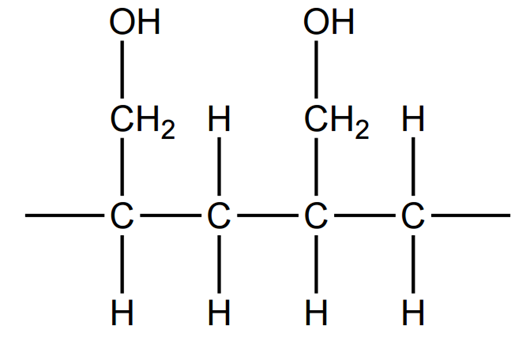
Reason for solubility in water : This polymer is likely to be water-soluble due to the presence of hydroxyl (OH) groups. The OH groups can form hydrogen bonds with water molecules, which increases the solubility of the polymer in water.
[2]
[ii]
Outline two ways that waste hydrocarbon polymers can be processed usefully, rather than being disposed of in landfill sites.
There are several effective methods for processing waste hydrocarbon polymers to avoid landfill disposal.
- Recycling: Polymers can be sorted, cleaned, and reprocessed into new products.
- Incineration: Waste polymers can be incinerated to generate heat. This heat can be used to generate electricity or provide heating.
- Feedstock recycling: waste polymers are chemically broken down into their basic chemical building blocks, which can then be used as raw materials (feedstock) for creating new products.
[2]
END OF QUESTION PAPER